HVDC Converter: Best Guide to HVDC Transmission System
HVDC Converter technology plays a pivotal role in modern power transmission, enabling efficient and long-distance electricity transfer. In this comprehensive guide, we will delve into the world of HVDC conversion, exploring its various facets, from the types of HVDC converters to control systems, efficiency, environmental impact, and future trends.
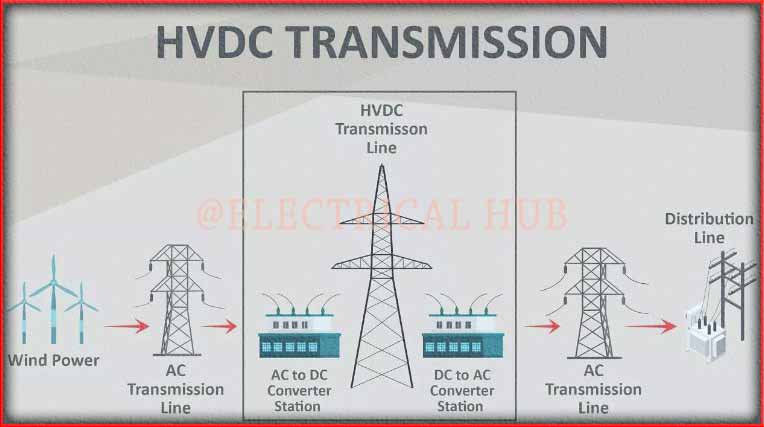
Read More About
What is an HVDC transmission system?
High-voltage direct Current is known as HVDC. It is a technique used to transmit electricity over great distances in the electrical power sector. HVDC systems transmit energy in a unidirectional flow of direct current (DC), as opposed to the more popular Alternating Current (AC) transmission, which periodically changes the direction of electric current.
Advantages of HVDC Transmission
Asynchronous AC grids can be connected thanks to HVDC converters, which also offer increased voltage stability and significantly reduced power loss during transmission. HVDC is an excellent choice for particular scenarios and applications in the electrical power sector because of these benefits.
Efficiency: When transporting electricity over vast distances, HVDC systems perform quite well. Compared to AC systems, they have reduced line losses, which means that less electrical energy is lost during transmission as heat. When transporting power across thousands of kilometers, this efficiency is quite helpful.
Reduced Power Loss: When AC transmission lines travel long distances, resistance in the cables causes large power losses. HVDC lines, on the other hand, experience less loss since they run at a constant voltage and transfer electricity as direct current, which encounters less resistance.
Long-Distance Transmission: HVDC transmission is a good choice for transferring power over long distances. Including underwater cables that connect continents or power transmission between remote locales, it may efficiently carry electricity over large geographic areas.
Voltage management: HVDC systems provide accurate voltage level management. As a result, they can be used to connect asynchronous AC grids that might operate at various frequencies. For grid synchronization, HVDC converters can change the voltage and phase angles as necessary.
Grid Stabilisation: By offering control over the electricity flow, HVDC can help to maintain grid stability. By dynamically managing the power flow and stabilizing voltage, it can aid in the prevention of grid disturbances and blackouts.
Grid Interconnection: HVDC converters enable the linking of various AC grids. This is crucial for exchanging power between regions or countries, enabling better utilization of renewable energy resources and improving grid reliability.
Renewable Energy Integration: HVDC technology is essential for integrating renewable energy sources into the current electrical system, such as offshore wind farms and remote solar projects. It enables the effective transportation of renewable energy from renewable energy generation facilities to urban centers.
Reduced Environmental Impact: HVDC transmission can help reduce greenhouse gas emissions and environmental impact thanks to its effectiveness and decreased power losses. In order to achieve sustainability and environmental goals, this is crucial.
Lower Electromagnetic Interference: When compared to high-voltage AC lines, HVDC systems emit less electromagnetic interference. This can make it more suitable for some applications by reducing interference with communication and control systems.
Capacity Expansion: By adding converter stations and transmission lines, HVDC systems may be expanded reasonably quickly. This scalability allows for future capacity upgrades to meet growing energy demands.
Applications of HVDC transmission system
Due to its distinct advantages and capabilities, High-Voltage Direct Current (HVDC) technology is used in a variety of electrical power industry applications. The following are some important uses for HVDC transmission systems:
Asynchronous AC grid interconnection: HVDC is frequently used to link two or more asynchronous AC grids that operate at various frequencies. This makes it easier to transfer power between areas or nations with incompatible grid frequencies.
Long-Distance Power Transmission: Transmission of electricity over very long distances with the least amount of power loss is possible using HVDC. It is frequently used to carry power from distant power plants (like hydroelectric dams) to metropolitan centers.
Submarine Cable Systems: Submarine cable systems are utilized to link continental landmasses, far-flung islands, and offshore wind farms. Because it reduces losses and enables effective power transmission over bodies of water, the HVDC transmission system is recommended for submarine cables.
Renewable Energy Integration: HVDC technology plays a crucial role in integrating renewable energy sources into the grid. It enables the efficient transfer of electricity from wind farms, solar installations, and other renewables to load centers.
Cross-Border Power Exchange: HVDC interconnections between neighboring countries allow for the exchange of surplus power. This enhances energy security, optimizes resource utilization, and supports electricity trading.
Grid Stabilization and Control: HVDC systems provide grid stability and control by regulating power flow, supporting voltage control, and enhancing overall grid reliability.
Islanded Grids and Remote Areas: In remote or islanded areas where access to reliable AC power is limited, HVDC can be used to connect these regions to the main grid or to transfer power between islands.
Underground Power Transmission: HVDC is employed for underground power transmission in densely populated urban areas where space for overhead lines is limited or aesthetically undesirable.
Back-to-Back Converter Stations: HVDC back-to-back converter stations are used to connect two nearby AC grids with similar frequencies while offering the advantage of isolation between them.
Electric Vehicle Charging: HVDC technology is utilized in high-power electric vehicle charging stations, enabling fast charging for electric cars, buses, and trucks.
Oil and Gas Industry: In offshore oil and gas platforms, HVDC is used for electrical power distribution, as it provides efficient and reliable power transmission over long distances.
Mining Operations: HVDC transmission systems are employed in mining operations, where power needs to be transported to remote mining sites efficiently.
Energy Storage Integration: HVDC transmission systems can be used to connect energy storage facilities to the grid, allowing for the efficient charging and discharging of stored energy.
Grid Modernization Projects: HVDC technology is often incorporated into grid modernization projects aimed at improving the overall reliability and efficiency of electrical grids.
Types of HVDC Converters
High-voltage direct Current (HVDC) converters are key components in HVDC transmission systems, responsible for the conversion between alternating current (AC) and direct current (DC). There are two primary types of HVDC converters:
Line-Commutated Converters (LCC):
Line-commutated converters are the traditional and older types of HVDC converters. They operate based on the natural commutation of power semiconductors, typically thyristors. Key characteristics and components of LCCs include:
Thyristors: LCCs use thyristor valves to control the flow of current. Thyristors are semiconductor devices that can only be turned on when a trigger voltage is applied and can only be turned off when the current passing through them falls to zero.
Natural Commutation: LCCs rely on the natural zero-crossing of the AC voltage to turn off the thyristors. This means that they can only convert AC to DC when the AC voltage passes through zero.
Limited Controllability: LCCs have limited controllability compared to Voltage-Sourced Converters (VSCs). They cannot change the direction of power flow (rectification to inversion) as quickly as VSCs, and they are not as capable of controlling the voltage and frequency on the DC side.
Applications: LCCs are still used in some HVDC projects, especially for long-distance, high-power transmission. They are known for their robustness and reliability.
Voltage-Sourced Converters (VSC):
Voltage-sourced converters are a more modern type of HVDC converter that utilize insulated gate bipolar transistors (IGBTs) as the switching devices. VSCs offer greater controllability and flexibility compared to LCCs. Key characteristics and components of VSCs include:
IGBTs: VSCs use IGBTs as their main switching devices. IGBTs allow for more precise control of the output voltage and current and can be turned on and off as needed.
Active Commutation: VSCs actively control the commutation process, meaning they can switch between rectification (converting AC to DC) and inversion (converting DC to AC) rapidly and independently of the AC voltage zero-crossing.
Advanced Control: VSCs offer advanced control capabilities, including the ability to regulate the voltage and frequency on the DC side, provide reactive power support, and dampen AC system oscillations.
Applications: VSC-based HVDC converters are commonly used in a wide range of applications, including connecting offshore wind farms, interconnecting grids with varying frequencies, and providing grid stabilization services.
While LCCs are still in use for certain applications, VSC-based HVDC converters have become the preferred choice for many modern HVDC projects due to their advanced control capabilities, faster response times, and greater flexibility in handling complex grid scenarios.
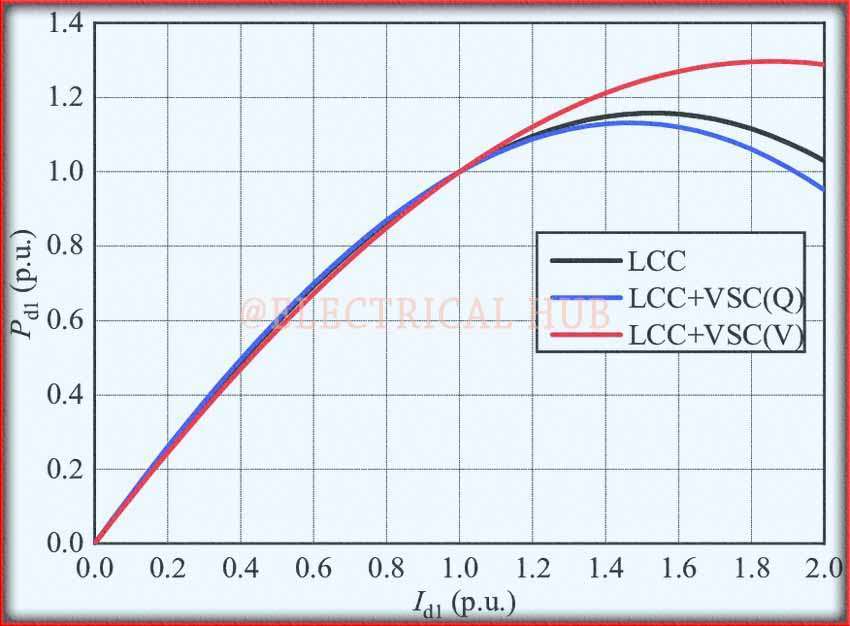
Components of HVDC Converters
Complex devices called High-Voltage Direct Current (HVDC) converters are used to switch electrical power between alternating current (AC) and direct current (DC). These converters are made up of a number of parts and systems that cooperate to provide dependable and effective power transmission. The following are the main elements of HVDC converters:
Converter Stations: Converter stations are the heart of an HVDC system. There are typically two converter stations in an HVDC link: one at the sending end and one at the receiving end. These stations house all the necessary components for AC-to-DC and DC-to-AC conversion.
Valve Halls: Valve halls contain the semiconductor devices used for power conversion. In Line-Commutated Converters (LCCs), these devices are thyristors, while in Voltage-Sourced Converters (VSCs), insulated gate bipolar transistors (IGBTs) are used.
Transformer: A transformer is used to step up or step down the voltage of the AC power before it is fed into the converter. Transformers are essential for matching the voltage levels between the AC grid and the converter.
AC Filters: AC filters are used to suppress harmonics and other unwanted frequencies in the AC power before it enters the converter. These filters help maintain the quality of power on the AC grid.
DC Filters: DC filters are used to smooth the DC voltage and reduce harmonics on the DC side of the converter. They ensure a stable and clean DC voltage output.
Converter Control System: The control system is a critical component that governs the operation of the converter. It includes hardware and software components responsible for regulating voltage, current, and power flow, as well as ensuring the safe and stable operation of the converter.
Cooling System: HVDC converters generate heat during operation, and efficient cooling systems are essential to maintain the proper temperature of the semiconductor devices and other components. Cooling methods may include air cooling, water cooling, or a combination of both.
DC Smoothing Reactor (Optional): In some HVDC systems, a DC smoothing reactor is used to further smooth the DC voltage and reduce ripple. This is particularly important in VSC-based converters.
Protection and Control Panels: Protection and control panels are responsible for monitoring the converter’s performance and ensuring safety. They include relays, sensors, and communication systems that detect faults and trigger protective actions.
Grounding System: A robust grounding system is essential for safety and to provide a path for fault currents in the event of a system fault.
Communication Equipment: Communication systems are used for data exchange between the two converter stations and with the overall control center. This ensures coordinated and synchronized operation.
Auxiliary Power Supply: Auxiliary power supplies provide power to various control, monitoring, and communication systems within the converter station. They are usually backed up by uninterruptible power supply (UPS) systems for reliability.
Monitoring and Diagnostic Systems: These systems continuously monitor the health and performance of the converter components, allowing for early detection of issues and preventive maintenance.
These components work together to enable the conversion of electrical power between AC and DC in HVDC converters. The choice of components and their design may vary depending on the specific HVDC technology (LCC or VSC) and the application, but their fundamental roles remain consistent in ensuring efficient and reliable power transmission.
Subscribe to our Newsletter “Electrical Insights Daily” to get the latest updates in Electrical Engineering. You can also Follow us on LinkedIn and Facebook to see our latest posts on Electrical Engineering Topics.
More Electrical Engineering Articles