Fault Current Distribution in Star Delta Transformer
Understanding fault current distribution in star delta transformer is critical for power system protection and design. Transformers are central components in any electrical distribution system, and their fault response behavior can affect overall grid stability. When a fault occurs in the system, the type of transformer connection plays a major role in how the current is distributed and managed. The star-delta configuration is widely used for this reason. It offers unique fault characteristics that engineers must account for during planning and protection.
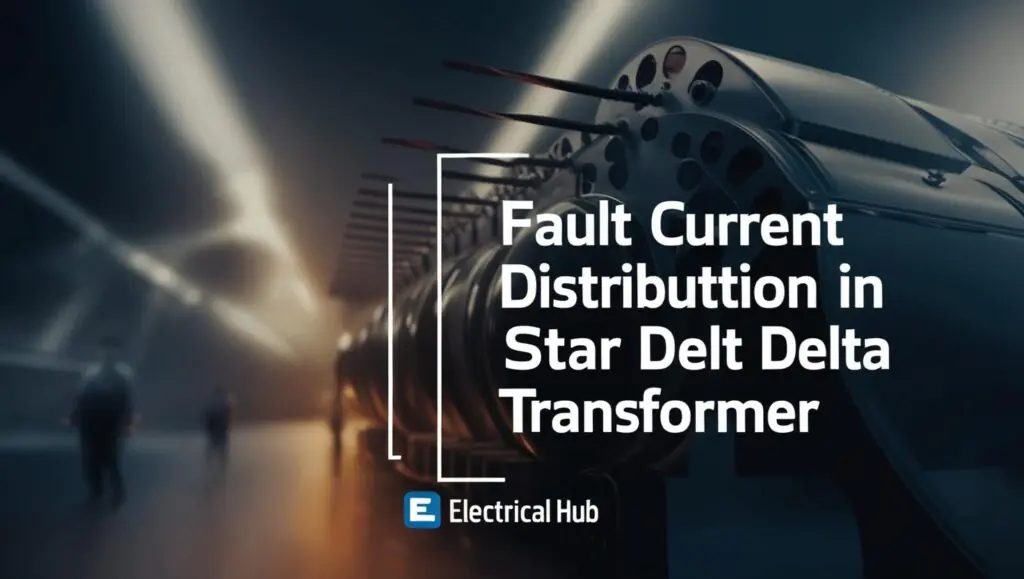
In this article, we will dive deep into how fault currents behave in star-delta transformers. We’ll break down the technical concepts into simple, short paragraphs and maintain a high readability score. You’ll also find helpful tables and practical examples along the way.
What Is Fault Current Distribution in Star Delta Transformer?
The term fault current distribution in star delta transformer refers to how electrical fault currents flow through a transformer that has its primary side in star (Y) connection and secondary side in delta (Δ) connection, or vice versa. This distribution is influenced by several factors such as the grounding system, type of fault, and transformer impedance.
When a fault happens on one side of a transformer, the way the current spreads into the other side depends on the winding configuration. A star-delta transformer behaves differently for line-to-ground, line-to-line, or three-phase faults.
Basic Operation of Star Delta Transformer
In a star-delta transformer, the primary winding is typically connected in star, and the secondary in delta. The star side allows grounding and is usually the high-voltage side. The delta side, often ungrounded, is on the low-voltage side.
This setup changes how fault currents return to the source. The delta connection provides a closed path for circulating currents, especially for unbalanced faults. Meanwhile, the star connection provides a neutral point that can be grounded.
This arrangement affects how much current flows during different faults, how quickly protection devices trip, and how voltage is restored.
Types of Faults and Their Impact on Distribution
Faults in a power system are broadly classified as symmetrical or asymmetrical.
- Symmetrical faults involve all three phases equally. These are rare but severe.
- Asymmetrical faults involve one or two phases and are more common.
Here’s how different types of faults affect fault current distribution in star delta transformer:
Type of Fault | Star Side Impact | Delta Side Impact |
---|---|---|
Single Line-to-Ground | High current due to neutral grounding | Fault may not reflect due to closed delta |
Line-to-Line | Moderate current, limited by impedance | Reflected clearly on both sides |
Double Line-to-Ground | High current, clear path through neutral | Circulating current in delta |
Three-Phase Fault | Symmetrical current, high magnitude | Same current in all three phases |
As the table shows, the delta winding can mask certain faults from appearing on the star side. This makes detection and fault current calculations more complex and system-dependent.
Technical Insight: Zero Sequence Currents
A crucial factor in fault current distribution in star delta transformer is the behavior of zero-sequence currents. These are currents that are equal in magnitude and phase in all three lines. They usually appear in line-to-ground faults.
In a star-delta transformer:
- Zero-sequence currents can flow in the star side if a neutral path exists.
- These currents cannot flow in the delta side because there’s no path for them.
However, the delta winding can support circulating currents within itself. These internal loops help balance the magnetizing currents, making the system more stable.
This is one of the key reasons engineers choose star-delta transformers in distribution networks. They help isolate and contain certain fault types.
Example: Fault on the Star Side
Suppose a single line-to-ground fault occurs on the star side of a transformer. The neutral provides a low-resistance path for the return current. The delta side does not experience this fault directly. However, due to transformer coupling, some unbalanced current may appear.
Here, the delta winding suppresses the propagation of zero-sequence components. Protection relays on the delta side may not detect the fault, making it essential to place primary protection on the star side.
The fault current of transformer in this case is determined by the impedance of the primary windings and the system grounding. If the system is solidly grounded, the fault current can be quite high.
Example: Fault on the Delta Side
Now consider a line-to-line fault on the delta side. The delta winding allows the fault current to circulate. The transformer transfers this unbalanced load to the star side.
Unlike zero-sequence currents, positive and negative sequence components do reflect to the star side. Relays and protection on both sides may respond.
The amount of current flowing depends on transformer impedance, source impedance, and fault resistance. These are key parameters in fault current calculations.
Transformer Impedance and Its Effect
Transformer impedance is critical in shaping the fault current distribution in star delta transformer. This impedance limits the amount of current that can flow during a fault.
The standard fault current calculation uses this formula:
I_f = V / (Z_source + Z_transformer + Z_fault)
Where:
- I_f is the fault current
- V is the system voltage
- Z_source is the source impedance
- Z_transformer is the transformer impedance
- Z_fault is the fault impedance
This fault current calculation formula is simplified but provides a good starting point. For real-world designs, symmetrical component analysis is often used.
Transformer impedance values are usually given as a percentage on the nameplate. For example, a transformer with 6% impedance limits the fault current to about 16.7 times full-load current.
How Star Delta Connection Limits Fault Propagation
The beauty of the star-delta configuration is its fault isolation ability. Here are a few protective advantages:
- Star grounding limits overvoltages and provides a safe path for ground faults.
- Delta winding contains unbalanced and third-harmonic currents.
- Zero-sequence currents from star do not pass into delta.
- Line-to-ground faults on one side do not always reflect fully to the other.
This behavior improves system stability and protection reliability.
Protection Scheme Considerations
Protection engineers must understand fault current distribution in star delta transformer to set relay parameters correctly. Depending on the location and type of fault, the current sensed by the relay may differ significantly.
Here are a few practical tips:
- Use differential protection on both sides for internal faults.
- Set ground fault relays only on the star side where the neutral is accessible.
- Install phase relays for line-to-line faults on both sides.
- In some cases, use restricted earth fault (REF) protection on the star side.
These choices depend on system design and fault sensitivity requirements.
Application in Industrial and Utility Systems
Star-delta transformers are common in medium-voltage to low-voltage step-down applications. Utility feeders, industrial substations, and motor control centers often use this connection.
In motor loads, the delta side helps with starting currents and suppressing voltage transients. The star grounding helps protect sensitive electronics during faults.
Fault current distribution in star delta transformer becomes even more critical in high-reliability environments such as data centers or hospitals, where quick isolation and restoration are essential.
Sample Fault Current Distribution Table
Below is a simplified distribution of fault currents in a star-delta transformer setup for a 33kV/11kV system with solid grounding on the star side:
Fault Location | Fault Type | Star Side Current (kA) | Delta Side Current (kA) |
---|---|---|---|
Star Side | SLG (Line-Ground) | 10.5 | 1.0 (Induced) |
Delta Side | L-L (Line-Line) | 5.0 | 5.5 |
Star Side | 3-Phase | 12.0 | 11.5 |
Delta Side | DLG (Double-Ground) | 9.5 | 9.0 |
This shows that while both sides see current during major faults, the star side generally carries higher magnitude due to neutral grounding.
Importance of Accurate Fault Current Calculations
Incorrect fault current calculations can lead to undersized circuit breakers, slow relay response, or even system instability. For transformers, these calculations must take into account:
- Connection type (star/delta)
- Grounding method
- System voltage levels
- Transformer and source impedance
Advanced simulation software often uses symmetrical component theory and real-time system models to improve accuracy.
Conclusion
The study of fault current distribution in star delta transformer is essential for designing reliable and protected electrical systems. This transformer configuration plays a unique role in isolating faults and controlling current flow between different voltage levels.
By understanding how zero-sequence, positive-sequence, and negative-sequence currents behave in star and delta windings, engineers can optimize protection schemes. The choice of grounding, relay settings, and fault detection methods all depend on these principles.
Follow Us on Social:
Subscribe our Newsletter on Electrical Insights to get the latest updates in Electrical Engineering.
#StarDeltaTransformer, #FaultCurrentDistribution, #TransformerProtection, #ElectricalEngineering, #PowerSystems, #TransformerFaults, #StarDeltaConnection, #ShortCircuitCurrent, #ElectricalSafety, #TransformerAnalysis, #PowerTransformer, #StarDeltaWiring, #ThreePhaseSystem, #FaultDetection, #CurrentDistribution