Wind Turbine Design With Example
Wind energy has emerged as a cornerstone of sustainable power generation, with wind turbine design playing a pivotal role in maximizing energy capture from this inexhaustible resource. The evolution of wind turbine technology represents a fascinating blend of aerodynamics, mechanical engineering, and environmental science.
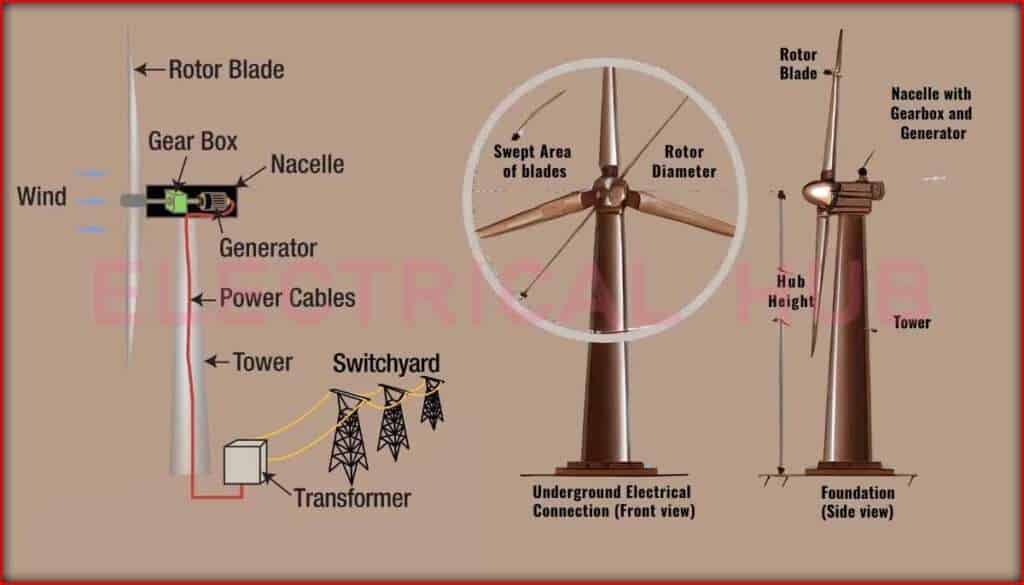
Table of Contents
Fundamentals of Wind Turbine Design and Operation
At its core, wind turbine design revolves around converting kinetic energy from moving air into mechanical power, which is then transformed into electricity. The fundamental power equation that governs wind turbine operation is:
P = ½ ρ A V³ Cp
Where:
- P represents the power output in watts
- ρ is the air density (typically 1.225 kg/m³ at sea level)
- A is the swept area of the rotor in square meters
- V is the wind velocity in meters per second
- Cp is the power coefficient (maximum theoretical value is 0.593, known as the Betz limit)
The relationship between wind speed and power output is cubic, meaning that doubling the wind speed results in an eight-fold increase in power output. This makes optimal wind turbine design crucial for efficient energy generation.
Key Components in Modern Wind Turbine Design
The rotor assembly forms the heart of any wind turbine design. It consists of precisely engineered blades attached to a central hub. Modern turbine blades typically employ airfoil profiles similar to aircraft wings, creating lift forces that cause the rotor to spin. The blade design process involves careful consideration of material selection, usually incorporating composite materials like fiberglass and carbon fiber for their excellent strength-to-weight ratio.
The nacelle houses the gearbox and generator, representing another critical aspect of wind turbine design. The gearbox increases the relatively slow rotation of the blades (typically 15-20 rpm) to the higher speeds required by the generator (around 1500 rpm). The design challenge lies in creating a robust system that can handle varying loads while maintaining efficiency.
Advanced Considerations in Wind Turbine Design
Modern wind turbine design incorporates sophisticated control systems to optimize performance across different wind conditions. Pitch control mechanisms adjust blade angles to regulate power output and prevent damage during high winds. These systems work in conjunction with yaw controls that rotate the entire nacelle to keep the rotor facing into the wind.
The tower structure, while seemingly simple, requires careful engineering in wind turbine design. It must support the entire nacelle and rotor assembly while withstanding dynamic loads from varying wind conditions. The tower height is optimized based on the wind shear profile at the installation site, with taller towers accessing stronger, more consistent winds at higher altitudes.
Engineering Calculations in Wind Turbine Design
The detailed design process involves several key calculations and considerations:
Tip Speed Ratio (λ) = (Rotor tip speed) / (Wind speed) λ = (ω × R) / V
Where:
- ω is the angular velocity in radians per second
- R is the rotor radius in meters
- V is the wind speed in meters per second
Optimal tip speed ratios typically range between 6 and 8 for modern three-bladed turbines. This parameter significantly influences the blade design and overall turbine efficiency.
Practical Wind Turbine Design: A Step-by-Step Engineering Example
Design Specifications, for Example, Wind Turbine
Let’s design a horizontal-axis wind turbine with the following target specifications:
- Rated power output: 100 kW
- Design wind speed: 12 m/s
- Installation location: Coastal area at sea level
- Type: Three-bladed, upwind configuration
Step-by-Step Wind Turbine Design Process
Step 1: Initial Power and Rotor Size Calculations
First, we’ll determine the rotor diameter needed to achieve our power requirements. Using the wind turbine power equation:
P = ½ ρ A V³ Cp
Where:
- P = 100 kW = 100,000 W (desired power output)
- ρ = 1.225 kg/m³ (air density at sea level)
- V = 12 m/s (design wind speed)
- Cp = 0.45 (typical for modern turbines)
Rearranging to solve for area (A): A = P / (½ ρ V³ Cp) A = 100,000 / (0.5 × 1.225 × 12³ × 0.45) A = 308.64 m²
The rotor diameter (D) can be calculated using: D = √(4A/π) D = √(4 × 308.64/π) D = 19.8 meters
Step 2: Blade Design Parameters
Now we’ll determine the optimal tip speed ratio (λ) and rotational speed:
For a three-bladed turbine, optimal tip speed ratio typically ranges from 6 to 8. Let’s choose λ = 7
Rotational speed (ω) can be calculated using:
ω = (λ × V) / R ω = (7 × 12) / 9.9 ω = 8.48 rad/s = 81.1 rpm
Step 3: Blade Geometry Calculations
For optimal performance, we’ll design the blade with varying chord length and twist angle along its length. Let’s divide the blade into 10 sections:
The chord length at any radius (r) can be calculated using: c(r) = (16 π R) / (9 λ² × r/R)
For example, at r/R = 0.3 (30% of blade length): c(0.3R) = (16π × 9.9) / (9 × 7² × 0.3) c = 2.84 meters
The twist angle (β) at each section is calculated using: β = arctan(2/3λ × R/r) – φ where φ is the airfoil’s angle of attack (typically 5-7 degrees)
At r/R = 0.3: β = arctan(2/(3 × 7 × 0.3)) – 6° β = 32.8 degrees
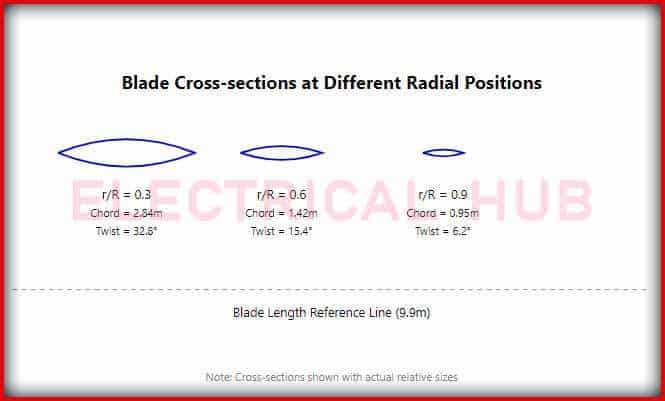
Step 4: Structural Design Considerations
For the blade material, we’ll use fiberglass-reinforced plastic with the following properties:
- Density: 1,800 kg/m³
- Ultimate tensile strength: 400 MPa
- Young’s modulus: 40 GPa
The maximum bending moment (M) at the blade root can be estimated using: M = ½ ρ V² A R Ct where Ct is the thrust coefficient (typically 0.7)
M = 0.5 × 1.225 × 12² × 308.64 × 9.9 × 0.7 M = 188,542 Nm
Step 5: Control System Design
The pitch control system needs to handle the following specifications:
- Maximum pitch rate: 8 degrees/second
- Operating range: -5 to 90 degrees
- Emergency feathering time: < 15 seconds
The control algorithm uses the following power curve regions:
- Below cut-in speed (4 m/s): Blades at 90° pitch
- Operating region (4-12 m/s): Optimal pitch for maximum Cp
- Above rated wind speed (>12 m/s): Active pitch control to maintain rated power
Step 6: Generator and Gearbox Specifications
Given our rotational speed of 81.1 rpm and required 100 kW output:
Gearbox ratio = Generator speed / Rotor speed = 1500 / 81.1 = 18.5:1
Generator specifications:
- Type: Doubly-fed induction generator
- Rated power: 110 kW (including margins)
- Operating speed: 1500 rpm
- Efficiency: 94%
Step 7: Tower Design
For our 19.8m rotor diameter, we’ll design a tubular steel tower:
- Height: 40m (approximately 2 times rotor diameter)
- Base diameter: 2.5m
- Top diameter: 1.8m
- Shell thickness: 20mm
Natural frequency calculation: f = (1/2π) × √(k/m) where:
- k is tower stiffness
- m is effective mass including rotor and nacelle
Performance Predictions
Based on these calculations, our wind turbine design should achieve:
- Rated power output: 100 kW at 12 m/s
- Cut-in wind speed: 4 m/s
- Cut-out wind speed: 25 m/s
- Annual energy production (at average wind speed of 7 m/s): approximately 280 MWh
- Overall efficiency: 42% at rated conditions
Safety and Maintenance Considerations
The design includes:
- Triple braking system (aerodynamic, mechanical, and electromagnetic)
- Lightning protection with receptors in blade tips
- Condition monitoring system for:
- Vibration analysis
- Oil particle counting
- Temperature monitoring
- Structural health monitoring
Cost Estimation
Based on current market rates:
- Rotor assembly: $45,000
- Nacelle components: $65,000
- Tower and foundation: $40,000
- Control systems: $25,000
- Installation: $35,000 Total estimated cost: $210,000
This detailed design example demonstrates the interconnected nature of various wind turbine components and the iterative process required to achieve optimal performance. Each parameter affects multiple aspects of the design, requiring careful balance and optimization.
Environmental and Safety Aspects of Wind Turbine Design
Environmental considerations have become increasingly important in wind turbine design. Engineers must account for wildlife impact, particularly concerning bird and bat populations. This has led to innovations in blade coloring, lighting systems, and even radar-based shutdown systems during heavy migration periods.
Safety features are paramount in modern wind turbine design. Lightning protection systems are integrated throughout the structure, while multiple braking systems ensure safe operation. The mechanical brake serves as a backup to the aerodynamic braking achieved through blade pitch control. Condition monitoring systems continuously assess structural health and performance metrics.
The foundation design varies based on installation location, with offshore wind turbine design presenting unique challenges. Offshore installations must account for wave loading, seabed conditions, and corrosion protection, often resulting in significantly different design approaches compared to onshore turbines.
Future Trends in Wind Turbine Design
The wind energy sector continues to evolve, with emerging trends focusing on larger turbines, particularly for offshore applications. Some designs now exceed 12 MW in capacity, with rotor diameters surpassing 220 meters. These massive machines require innovative solutions in materials science and structural engineering.
Digital twin technology is revolutionizing wind turbine design and maintenance. These virtual replicas enable engineers to simulate different operating conditions and optimize performance parameters before physical implementation. Machine learning algorithms are increasingly employed to predict maintenance needs and adjust operating parameters for maximum efficiency.
The integration of energy storage solutions is becoming an important consideration in wind turbine design. Some concepts incorporate hydrogen production facilities or battery storage systems directly into the turbine structure, addressing the intermittency challenge inherent to wind power.
As wind energy continues to play a crucial role in the global transition to renewable energy, wind turbine design will remain at the forefront of technological innovation. The continuous refinement of existing designs and the emergence of new concepts promise even greater efficiency and reliability in harnessing the power of the wind.
Worth Read Posts
- Wind Energy
- Smart Grid
- Wind Turbine Design Ideas
- What Are 555 Timers
- DL2450 vs CR2450
- Fading and Attenuation
- CR2032 vs CR2450
- CR2450N Battery
- CR2450 Battery Equivalent
Follow us on LinkedIn, “Electrical Insights,” to get the latest updates on Electrical Engineering.